The Lives of Bees, T.Seeley, Chapter 9
- Gemma
- Oct 1, 2020
- 28 min read
Updated: Oct 5, 2020

New episode of the podcast is up! You can listen on Podbean, or wherever you get your podcasts.

Homestead news:
The chickens continue to moult, and I’m down to 1-4 eggs a day from 8 hens (I don’t include Agatha and Bubbles since they don’t lay anymore). This is my favourite time of year when it comes to the chickens because I found Bobette in the Fall, and so did most of my prepping through Fall and early Winter. The season makes me think of chickens and makes me nostalgic for all the discoveries of those days! I learned so much in a short time and everything was a new adventure. I like to sit out with the girls more when I can now, and I enjoy watching them go about their chickeny business.
Agatha is continuing with the pain meds and seems comfy. I’ve also noticed a dramatic decrease in lice since her Frontline application, and I am cautiously optimistic that this will continue. I’m going to double check how regularly I can treat with this. For those curious, the main ingredient in Frontline is fipronil. A cursory reading of the literature indicates that you can repeat the treatment but I have not found for how long yet without it becoming problematic.
Speaking of Agatha, she continues to run when she sees me coming! I have to sneak up on her these days as even the highest value treats will not make her come out. I think she is just done with being ‘messed’ with; be it treating for lice or giving her medication. Poor old gal just wants to be left alone! I wish I could make her understand that this is all for her benefit.

Hive news:
I’ve had some frustrating days in the apiary lately, to be honest. The Fall flow is here and I anticipated less aggression and robbing behaviour from my colonies. Thankfully, aggression is down but the robbing continues. I’m already behind on mite tests due to being unable to get in the colonies without them becoming insanely spicy, and now I have struggled to get in fast enough to get everything done without causing a dangerous robbing situation. I’ve found cooler mornings to be the best time; the bees are grumpy, yes, but I have more time before other bees realise the hive is open.
Looking at my notes, I basically didn’t go in from mid August to late September due to the robbing and aggression. In hindsight, I probably should have been feeding during this time due to the hard dearth but I was very worried this would increase the robbing behaviour and cause more destruction. My nucleus colonies are especially vulnerable due to their smaller size. I might consider establishing future nucleus colonies in a completely separate area, far away from the larger ones.
Mite testing results are quite interesting this year. Hive #1 (OH queen) tested as 8/300, which is within the expected Fall range, though does require treatment. Hive #2 (Southern US queen) had 1:300 when I tested 09/12 but just 15 days later their count had soared to 12:300. Hive #3 (Saskatraz daughter queen) also had a count of 12:300. Hive #4 (Saskatraz queen) had a count of 9:300. Hive #5 (which was previously nuc #3, and has OH or Sask genetics) tested as 12:300. I’m leaning to this one being Sask because they still have a lot of drones, which is a trend I am noticing among all my Sask colonies.
Nucleus colonies are a mixed bag. Nuc #1 (Ohio genetics) is looking good; nice brood build up, still have honey stores despite the dearth, and mite count is okay at 6:300. Nuc #2 (unknown genetics; could be OH/Southern/Sask) is a mess. The queen, who was my favourite from the nucs, has swarmed and there’s a new, smaller queen in there. She might even be a virgin, which is bad news since this is not the best time of year for mating. The colony also looks shoddy; spotty brood pattern and not a lot of stores. The mite count is dangerously high at 19:300 bees. I don’t know if they will survive and I almost suspect that they have been robbed, which would explain the lack of stores and potentially the high mite load. I’m going to treat and feed the hell out of them and hope for the best!
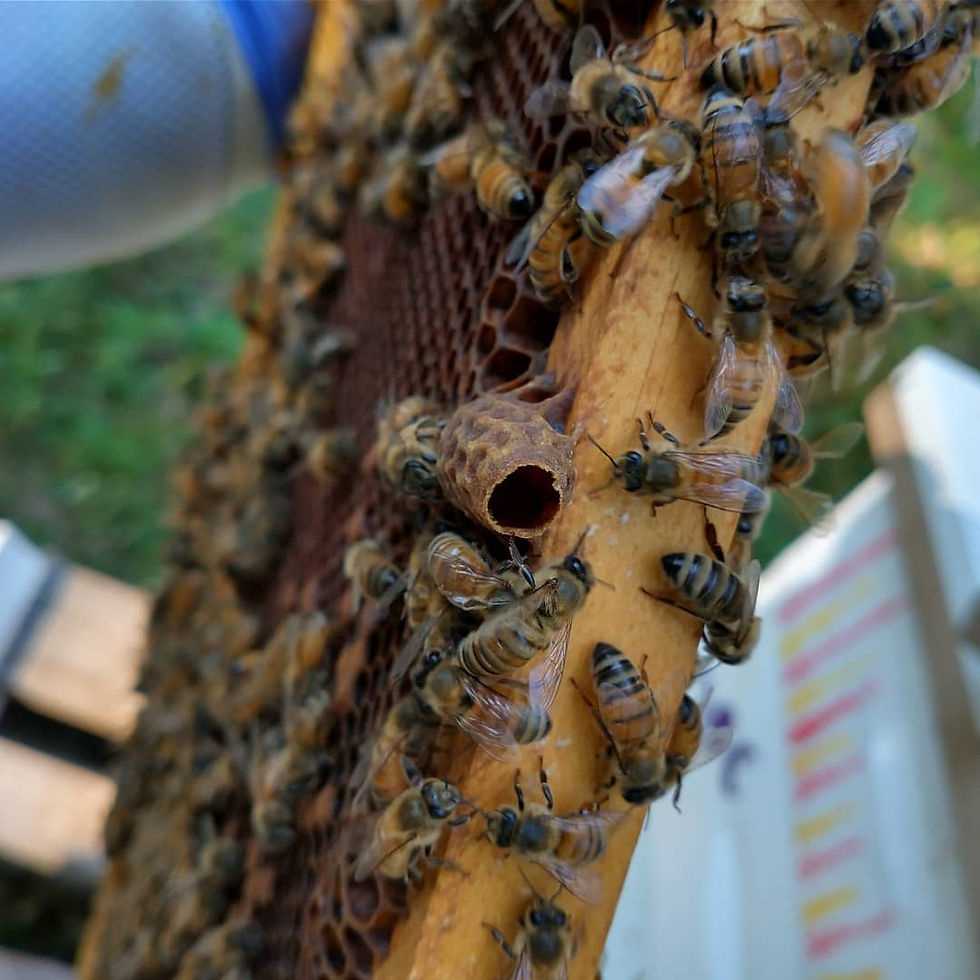
Thinky thoughts: so far, my Ohio genetics have the lowest mite count, which is an interesting discovery. Next in line is my Sask mother queen but her daughter queen colonies have high levels, which I admit to being surprised about since I partially got this lineage due to their mite resistance.
Robbing. It’s been bad this year. Because of the long dearth? Having more colonies in the apiary? Or are my Sask bees the robbers? As we saw in Seeley’s ‘The Lives of Bees’, robbing facilitates disease and mite transmission. Could my Sask bees be bringing home mites and therefore increasing their colony mite counts? I have moved one of my hives as it was much closer to another than I liked (but I lacked a hive stand at the time and had to make do). If I add any new colonies next Spring, I will be spacing them FAR apart.

That jump in mites in hive #2 really makes me wonder if there was a ‘mite bomb’ in my area; a hive that reached unsustainable levels and perished. If my hives robbed them, they would bring the mites home along with the stolen goods. Something to consider.
Robbing. It’s been bad this year. Because of the long dearth? Or are my Sask bees the robbers? As we saw in Seeley’s ‘The Lives of Bees’, robbing facilitates disease and mite transmission. Could my Sask bees be bringing home mites and therefore increasing their colony mite counts? I have moved one of my hives as it was much closer to another than I liked (but I lacked a hive stand at the time and had to make do). If I add any new colonies next Spring, I will be spacing them FAR apart.
Local/US genetics. My Sask queen was open mated in California so her offspring have a mix of genes; they’re not 100% Saskatraz. This could affect their mite resistance. Similarly, my Sask daughter queen has even less Sask genetics and therefore her offspring will show differing abilities. Honestly, if they overwinter well, I’ll be happy!
Drones. All the Sask colonies have more drones later into the year, and one colony is even still producing drones at this time. Drone cells are preferred by varroa mites so this is likely a contributing factor to their higher levels.
Mite count vs resistance. My understanding of mite resistance in bees is that it is demonstrated predominantly in behavioral traits such as leg biting, increased grooming, opening brood cells, or simply chewing holes into brood cells to detect mites. Is it possible, though, that mite counts could be higher in a colony that is less susceptible to disease? I’m just spitballing here but I am very interested to see whether these Saskatraz bees can handle higher mite loads without deleterious effects. I can’t see why they would but I’m open to the possibility.
Treatments used: so far, I’ve used Apivar. This was my treatment option last year and I had good results with it. The main ingredient is Amitraz, and it’s supposed to have a mite kill rate of 99%. I’ll also be testing Apiguard this year on a few colonies. The active ingredient in that is Thymol, which is a naturally occurring substance derived from thyme. Both these treatments require that honey supers be taken off beforehand, and this was a huge pain in my ass. It also did not help with the robbing situation!! I chose these treatments after carefully reading about how they work and any potential side effects. Both had the lowest rates of queen and brood fatality that I could find, and also did not involve equipment like a vaporizer and appropriate protective equipment (such as that needed when using oxalic acid). That said, next year I am leaning towards Formic acid as a treatment since honey supers can be left on, and maybe oxalic acid dribble method in the Spring as an initial treatment before honey supers go on. We shall see! Either way, I should note that since I took the honey supers off, I’m currently feeding my hives a 2:1 syrup as well as Bee Pro Patties.
*
Onwards to the book review!
Chapter 10: Colony Defense
Life consists with wildness.
The most alive is the wildest.
-Henry David Thoreau, “Walking”, 1862
“Every living system faces a legion of predators, parasites, and pathogens, each of which is equipped with a sophisticated tool kit for penetrating the defenses of its prey or host. In the case of a honey bee colony, there are several hundred species, ranging from viruses to black bears, whose members are forever trying to breach the bees’ defenses.”
What makes a honey bee colony prone to predation?
Honey stores: a valuable sources of calories for insects and mammals alike
Brood and adult bees: good source of protein
Nest temperature: maintained at a toasty level that allows bacteria, fungi, and viruses to thrive
Stationary nest: bees invest a great deal in building comb and then filling it with brood and food stores, making fleeing during predation difficult/impossible. Only a very great danger could make them abandon the nest. Instead, they stay and defend it in a myriad of ways.
Just as we see with other wild animals, honey bees live with endemic infections of parasites and pathogens that they have evolved to survive. If a colony undergoes environmental stress, however, this delicate balance of host and parasite/disease is unbalanced and the colony can fail. Think about the role of stress on the immune system of many animals (including birds and reptiles): stress can lower the immune system defenses allowing a parasite or pathogen to surge in numbers. In a similar way, we see that some beekeeping practices provide undue stress on the honey bee and lowers its ability to fight off disease.
One example of this would be American foulbrood and chalkbrood. In nature, a wild honey bee colony will clean old comb after winter. In our managed hives, old comb is often stored away from the bees and given back in the Spring, thus potentially reintroducing the aforementioned pathogens back into the colony.
The classic example, and most problematic, is that of the transmission of the varroa mite. This began in the late 1800s, when Russian beekeepers began exporting the western honey bee (apis mellifera) from Europe to the Primorsky region of the Russian Far East. This placed the western (European) honey bee in close proximity to colonies of the eastern honey bee (apis cerana). This allowed a host shift of the ectoparasitic mite Varroa jacobsoni from the eastern honey bee to the western. Over time, this mite evolved into the nasty Varroa destructor we know today, and which has caused untold damage to our colonies of the western honey bee. Since the varroa mite feeds on the fat bodies of bees (note: Seeley says the blood of bees since this book was published before the discovery by Dr Samuel Ramsey that varroa actually feeds on the fat bodies of bees but the transmission of viruses is the same), viruses are easily transmitted from the mite to the bee, as well as between colonies. This transmission of viruses and disease has been one of the greatest effects of the varroa infestation, leading to virulent viruses such as the Deformed Wing Virus.
This chapter looks at the differences in how wild and managed colonies of honey bees are affected by disease and parasites. Most tellingly, we will see that, despite lack of human intervention when it comes to pathogens, wild colonies demonstrate greater success in colony defense than our managed apiaries.
Living Without vs With Treatments for Varroa Destructor
Treating our colonies with miticides has been common practice since the 1970s in Europe and the 1980s in North America. These treatments have been essential to the survival of colonies battling the virulent varroa mite. However, in the last 10 years or so, beekeepers and researchers have reported colonies of honey bees that appear to be thriving without the use of miticides.
Honey bee colonies in the Primorsky Kray region of Russia where the host shift of Varroa jacobsoni originally occurred, leading to the evolution of Varroa destructor, were naturally the first effected by this new parasite. Colony loss was huge but, over time, natural selection led to colonies that demonstrated resistance to the varroa mite. The resistance mechanisms of these bees were studied by Thomas E. Rinderer at the Honey Bee Breeding, Genetics, and Physiology Research Laboratory in Baton Rouge, Louisiana. In the late 1990s, studies indicated that these bees demonstrated an increased ability to groom varroa mites off their bodies, as well as removing infested pupae from their cells (and there was some indication that they might also be biting the legs off the varroa). As a result, varroa mite infestation grows far slower in these Russian colonies compared to US ones (or really any other population that has not undergone natural selection for resistance).

Although the Russian bees prove that Apis mellifera can evolve over time to develop resistance to the varroa mite, this process of evolution was not recorded and so it cannot be said how quickly these resistant traits developed. A study in Sweden, however, demonstrates just how swiftly resistance can evolve in our beloved honey bee. Led by the late Ingemar Fries, the goal of the study was to see whether varroa mites “will eradicate European honey bees in an isolated area under Nordic conditions, where no mite control or swarm control of honey bee colonies are implemented”.
In 1999, the study began. The researchers located the experiment on the southern end of Gotland, a 3200 square kilometer/1200 square mile island in the Baltic Sea. 150 genetically diverse colonies were distributed among 7 apiaries, and each colony was housed in a two-story Swedish hive (similar to the Langstroth hive). Once moved to the locations, each colony was left unmanaged, with the exception of feeding before winter if honey stores were insufficient. Queens were also marked so that queen turnover (via swarming or supersedure) could be recorded.
Originally, these colonies did not have varroa mites so the researchers introduced them by giving each colony 1000 bees from mainland colonies that were heavily infested. The colonies were checked 4 times a year to assess winter survival chances, colony size in early Spring, colony swarming, and mite infestation level in late October. Bait hives were put up to catch any swarms, and these were housed in vacant hives within the apiaries.
Going into the first winter of the study, mite infestation levels were low for all colonies, and the colony mortality rate over winter was also low at around 5%. The colonies were thus strong enough the following summer (2000) to swarm in high numbers, keeping the colony numbers at the apiaries stable. By October of that same year, the mite levels had risen and winter mortality increased to nearly 30%. In the summer of 2001, there was noticeably less swarming, and mite levels continued to rise through to October. That winter, colony mortality was almost 80%.
In 2002, the third summer of the study, there were few colonies still alive, and those were so weak that they did not swarm. By October, only 21 colonies remained, and they had high mite infestation levels, and continued to do poorly through the winter (mortality rate of 57%). By the summer of 2003, there was some improvement. Although only 8 colonies remained, 1 was strong enough to swarm, and the average mite level had begun to decline. Even better, that winter the mortality rate was just 12%. This trend continued and, in the summer of 2004, more than half the colonies were strong enough to swarm, the mite levels were their lowest yet, and their winter mortality was 18%. So, within just 5 years, we can see how natural selection allowed for these honey bee colonies to increase their resistance to varroa!
Since the Spring of 2005, the remaining colonies from the study have been left alone, and were monitored over the next 10 years (through 2015) to see how they would fare. It consisted of 20-30 entirely self-sustaining colonies.

Sadly, the genetics of the bees in this experiment were not studied so it’s impossible to examine what changes may have occurred to produce these survivor bees. However, comparing the Gotland survivor bees to those on the mainland showed that the Gotland bees had an 82% lower rate of mite population growth, regardless of the mites source (from the mainland or the Gotland population). This latter point is important to note because it indicates that the ability to survive the varroa infestation was due to an increased resistance in the bees, as opposed to reduced virulence of the mites.
Seeley points out that there are just two stages at which honey bees can effectively take action to decrease the reproductive success of varroa mites:
When the female mites are moving around (and feeding) on adult bees (the phoretic stage)
When the female mites are sealed in brood cells containing pupae (the reproductive stage)
No evidence was found to indicate that the Gotland survivor bees were attacking mites by biting off their legs and antennae, thus disrupting them at stage 1. This leaves stage 2: when the mites are inside the sealed brood, which led to the belief that the Gotland bees were adept at locating the mites within the cell. Further support of this comes from examining the reproductive success of mites found in the Gotland colonies. Around 50% of these mites produced viable and mated daughter mites, compared to 80% of the mites examined in the mainland colonies. Recent studies have shown that uncapping and recapping a brood cell with a mite inside is effective at reducing the reproductive success of the varroa mite. Therefore, it can be assumed that the Gotland survivor bees have evolved greater Varroa Sensitive Hygiene (VSH) in this area; detecting the mites in cells and acting in a way to disrupt their reproductive process (uncapping and recapping the brood cell, or removing the pupae).
Interestingly, the Gotland colonies tend to be smaller, swarm more frequently, and raise fewer drones; all of which can aid in reducing mite infestation. Smaller colonies mean less brood to parasitize and, arguably, greater success in grooming each other; swarming causes a natural brood break; and drones are the preferred host cells for the varroa mite. All of these factors likely contribute to these survivor bees’ success.
Seeley likens these Gotland survivor bees to the wild colonies he has studied within the Arnot Forest. As stated in chapter 2, Seeley first discovered varroa in his own colonies in 1994. Just 10 years later, in 2004, he discovered that the wild colonies in the area already demonstrated traits that led to mite resistance. So again we can see a rapid evolution of completely untreated and unmanaged colonies.
In 2011, Seeley again decided to examine the level of varroa mite infestations in wild colonies of the Arnot Forest. With the help of a Cornell student, Sean Griffin, he conducted a third survey of the wild colonies, using the same bee-lining methods to locate them as he had done previously in 1978 and 2002. Seeley’s first goal was to locate as many wild colonies as possible and collect a sample of 100 bees from each. His second goal was to find the nearest apiaries outside of the Arnot Forest and collect the same sample size from them as well. Genetic analyses of the samples were conducted by Seeley’s colleagues, Deborah A. Delaney and David R. Tarpy. The goal was to learn whether the wild colonies were self-sustaining or if they survived in part due to immigration from the managed (and treated) colonies outside of the forest.
From late July to September, Seeley and Griffin located a total of 10 wild colonies, and collected the 100 worker bee sample from each. They then looked for managed colonies within 6 kilometers/3.7 miles of the forest’s boundaries, finding just two, with each containing about 20 colonies. One was located just 1km/0.6 miles from the southwestern boundary line of the Arnot Forest, while the other was 5.2km/3.2 miles from the forest’s northeastern corner. Both belonged to the same owner, who was a commercial beekeeper. He gave his permission for the 100 worker sample to be taken from 10 colonies at each apiary location.
The genetic analysis of the sample indicated a large difference between the wild colonies and the managed ones, and demonstrated that even the closest managed colonies had little influence on the genetics of the wild colonies.
In 2011, Seeley was visited by a former Cornell student, Alexander (Sasha) Mikheyev, who learned about the project and asked whether Seeley had any samples of bees collected before the arrival of varroa mites to the area. Luckily, Seeley did still have bees that were collected in 1977. Some of these bees had been collected via bait hives placed within the forests, while others were sourced from wild hives found in trees, barns, and farmhouses south of Ithaca. In order to conduct a proper comparison, Seeley therefore needed to collect current bees from similar locations. In just a few days, he was able to get samples from 22 colonies living in these types of nest environments. Ultimately, Seeley gave Sasha 64 samples; 32 of which were collected 20 years before the arrival of varroa, and the remaining 32 collected 20 years after.

So what did the genetic analysis reveal? Some rather nifty findings!
First, the mitochondrial DNA (DNA passed from mother to offspring) showed a dramatic loss in diversity between 1997 and 2011. In fact, nearly all the old mitochondrial lineages were now extinct. Of those lineages that had survived, none were found in the commercial stocks of bees. This demonstrates that the wild colonies went through a genetic bottleneck sometime between 1977 and 2011. It also indicates that the wild colonies suffered a collapse in population after the introduction of the varroa mite but enough survived that the 2011 colonies can be traced back to just a small number of queens.
Looking at the nuclear DNA of the bees revealed that since 1977 there has been some introduction of genes from Africa, probably through colonies moved by beekeepers from Florida to New York. The results also showed significant changes in the bees genes; about half of which relate to the bees’ development. This indicates that the surviving colonies developed resistance mechanisms that are in some part due to changes in the development programs of their members. Interestingly, the bees sampled in 2011 are consistently smaller than those from 1977.

In 2015, one of Seeley’s students, David T. Peck, decided to investigate the mechanisms of mite resistance of the bees living in the Arnot Forest. As mentioned previously, intervention can occur during the mite’s phoretic phase (when they are moving about and feeding on adult bees), and during the reproductive phase, which is when the mite is sealed in a brood cell with the bee pupae. In the phoretic phase, mites can be groomed from bees and then damaged in some way, such as chewing off their legs to immobilize them, or biting off their antennae, which disorients them and has a similar effect, In the reproductive phase, bees can uncap the mite infested cells and remove the pupae inside, or simply uncap and recap the cells, which also disrupts the mites reproduction (known as VSH; varroa sensitive hygiene).
David T. Peck started his study by capturing swarms using bait hives, which were then moved into an isolated apiary outside of the forest. He then examined the captured bees for signs of grooming and chewing of the mites, as well as signs of cell uncapping. When compared to bees kept in a control colony (sourced from outside the Arnot Forest and from managed hives), Peck found that the wild Arnot Forest bees showed a greater grooming and chewing response, as well as a greater propensity for VSH. In fact, he found that the wild colonies had uncapped/recapped as much as 40% of their brood cells, which is dramatically higher than what was seen in the control colony.
Seeley points out that what is most critical here is that the wild colonies in the Arnot Forest display multiple behaviours that support varroa resistance:
“In short, they are deploying a diverse set of behavioral resistance weapons against Varroa destructor, not just a single silver bullet. Bee breeders, take note.” Pg. 258
Living With Colonies Far Apart vs Close Together
One of the greatest changes humans have inflicted on honey bee living is our propensity to keep their hives clustered closely together. In the wild, we have seen that colonies are spaced far apart, often greater than 1 kilometer (0.6 miles) from another colony. In our apiaries, we often line our hives up next to each other, sometimes only inches apart. This closeness of living increases the risk of robbing behaviour, disease transmission, and even reproductive issues such as a virgin queen returning to the wrong hive (and subsequently being killed).
By keeping our colonies so close together, we have also inadvertently sped up the evolution of extremely virulent strains of parasites and disease. Through horizontal transmission (the spread of disease between unrelated colonies) of disease agents, we now must contend with strains of pathogens and parasites that have evolved rapidly in order to boost their chance of reproductive success. Since the pathogens and parasites reproduce at a much faster rate than the bees, they are able to basically out compete the honey bees in this area, leaving the bees to play evolutionary ‘catch up’. Three good examples of this phenomena are varroa mites, American foulbrood, and the deformed wing virus.
Horizontal transmission of disease can occur due to beekeeper management, such as when frames are taken from one colony and given to another (a common practice for boosting a weak colony, aiding in queen rearing, etc). But it also occurs due to drifting, which is what we call it when an adult bee returns to the wrong hive by accident. Close proximity of colonies increases this process, especially when hives are positioned in a line so that all the entrances face the same direction, side by side. In this configuration, more than 40% of bees can drift to another colony, which readily increases the spread of disease transmission.
Seeley decided to conduct an experiment to examine how the spacing of colonies can affect disease and pathogen transmission. In June 2011, he established two groups of 12 small colonies in a designated natural area owned by Cornell University. One group had the hives arranged in a row, facing the same direction, and with less than 1 meter/3 feet between them. The colonies in the other group were dispersed throughout a long field so that the space between them averaged 34 meters/110 feet.
For both groups, the hive body was the same: 2 deep Langstroths hive bodies. To measure the amount of drift between the colonies, Seeley was remarkably cunning. He installed 10 of the 12 colonies (for each group) with a Golden Italian queen. These queens contain the Cordovan gene, which is expressed through a lack of melanin aka the dark colouring that we usually see on the honey bee. What this means is that any drones produced by such queens will not be the usual dark brown/black in colour but instead a bright yellow. In the remaining 2 hives for each group, Seeley installed a Carniolan queen, which means that all the drones in these colonies will have the dark brown/black colouration. These Carniolan colonies were placed in the center of their group.
Each individual queen was labeled with a paint dot to allow tracking of queen turnover via swarming or supersedure. During the 2 year study period (June 2011 to May 2013), none of the colonies were treated for varroa mites, nor were they managed beyond monthly inspections from May to October. All colonies started as 2 frame nucleus colonies and so spent the summer of 2011 building up size and strength. By the time winter of 2011 arrived, all colonies appeared to be in good health.

In the summer of 2012, Seeley noticed some key differences between the colonies crowded together and those dispersed widely apart. 7 out of 12 colonies in each group swarmed but those in the crowded apiary had poor success in requeening; only 2 were successful. In comparison, the dispersed colonies had a success of 5 out of 7. Seeley postulates that this lack of success was due to young queens returning to the wrong colony in the crowded apiary and subsequently being killed. In fact, he twice found a dead queen lying outside of a colony entrance.
He also noticed an increase in drifting drones in the crowded colonies. In two separate counts made in September 2011 and again in April 2012 (before swarming occurred), Seeley found that 46% (September) and 56% (April) of drones flying into the black Carniolan colonies were bright yellow! So the Cordovan drones had drifted into the Carniolan colonies. In the dispersed group of colonies, this level of drifting was dramatically lower at just 1-2%. It’s important to consider that this level of intercolony mixing seems likely to also occur with worker bees, especially if we consider that workers often return to the hive with valuable resources and are thus less likely to be turned away. In the dispersed
In later summer of 2012, varroa levels soared in the 2 crowded colonies that had swarmed and successfully requeened, compared to those 5 that had also swarmed in the dispersed colonies. All of the 7 colonies that had swarmed had low mite counts in June and July but only the 5 dispersed colonies maintained these low levels as the year progressed.
When the experiment ended n May 2013, Seeley found that none of the 12 crowded colonies had survived. In comparison, 5 of the dispersed colonies were not only alive but also thriving. This is a dramatic result but Seeley cautions that it is just one small scale study in one particular geographical area so, although not definitive, it is a valuable step in better understanding how the distance between wild colonies likely aids in their survival.
Living in Small vs Large Nest Cavities
In chapter 5, we learned that the wild colonies of the Arnot Forest live in tree cavities with an average volume of 30-60 liters (8-16 gallons), which is far smaller than the hives most US beekeepers use for their colonies that average 120-160 liters (32-42 gallons). We do this because we want to encourage the bees to make more honey for our consumption. Providing an extra 100+ liters (or 26 gallons) of space enables the bees to store as much as 50 kilograms (100lbs) of honey.
Spacious hives also help decrease the risk of swarming, In chapter 7, we saw that a prime (first) swarm leaves with nearly 75% of the colony workers, which provides the bees with enough hard workers to build the comb needed in their new home but means a much smaller colony (that needs to raise a new queen) left behind for us, which in turn means a dramatically smaller honey harvest (if any).
So although large hives are advantageous for beekeepers, they are less beneficial to the bees, mainly because they hinder the bees natural reproductive process via swarming. After the arrival of varroa mites, this issue has been compounded because a large colony of bees is likely to lead to a large varroa infestation. Seeley himself demonstrated this via a study that compared to groups of hives; one group housed in small hives, and one in large. With the help of 2 of his students, J. Carter Loftus and Michael L. Smith, Seeley set up two groups of 12 hives, each apiary location being 60 meters/200 feet apart. In one group, each colony was housed in a small hive (42 liter/11.1 gallon) consisting of one deep, 10 frame Langstroth hive body. In the other group, colonies were established in a large hive (168 liter/44.4 gallon), which consisted of 2 deep Langstroth hive bodies for brood, and 2 deeps for honey storage. These larger hives were thus set up as one would if they were hoping to maximize honey production, including the removal of any queen cells found during inspections.
These 24 colonies were established in May 2012 and studied over the next 2 years. Once a month, from May through to October, the team tracked each colony’s brood and adult bee populations, mite levels, signs of disease, and signs of swarming/queen turnover. Honey production was also recorded. All 24 colonies started as 5 frame nucleus colonies with young queens purchased from a Californian breeder. No miticide treatments were administered during the entire 2 year study.
Seeley and his students predicted that the small hive colonies would do better over the 2 years as their smaller size would mean a greater chance of swarming, which would in turn mean a lower mite infestation due to the decrease in population and subsequent brood break. A previous study had shown that a prime scwar, can lead to a 35% reduction of varroa mites. This makes sense when we consider the fact that around 70% of a colony’s adult bees leave with the swarm, and that 50% of varroa mites live on the adults. We have also seen how brood breaks can help mitigate varroa levels as they deprive the mite of the capped cell needed for the reproductive phase of their life cycle.
When the experiment started in 2012, the average colony populations were equal amongst the group but over the summer of 2013, there was a marked divergence in numbers. On average, the colonies housed in the small hives did not grow beyond 10,000 bees, compared to 30,000 in the large hive colonies. The average level of varroa mite infestation also started the same in both colony groups but then changed again in summer 2013. The small hive colonies maintained a roughly steady level of 2 mites per 100 bee (considered a low level) until September, when it suddenly spiked dramatically (to 6 mites per 100 bees) before dropping. The large hive colonies showed consistent, high levels of mites (6:300) at a steady increase over time (see diagram). What’s particularly interesting is that 3 of the small hive colonies experienced extremely dramatic spikes in mite counts in September, reaching levels of 15-17 mites per 100 bees!

What contributed to these different varroa infestation levels? First, in 2013 nearly all (10 of the 12) small hive colonies swarmed, while only 2 of the large hive colonies did so. This likely explains why the mite levels in the small hive colonies were so low in 2013, as 10 of them experienced both the removal of varroa on adult bees that went with the swarm, as well as a brood break. It is not surprising then to see that the large hive colonies suffered higher winter mortality (10 out of 12 colonies lost) compared the the small hive colonies (only 4 of the 12 perished).
But what to make of that shocking varroa mite count found in 3 of the small hive colonies in September 2013? This event coincided with the collapse of 1 of the large hive colonies housed only 60 meters (200 feet) away. When Seeley examined the collapsed colony, he found a pile of dead bees in front of the hive, and almost no bees, no brood, no stored honey, and very few mites inside. The floor of the hive was littered with dead bees (most with shriveled wings indicative of deformed wing virus) and the wax flakes that indicate robbing had occurred of the food stores. Clearly, this colony had collapsed due to its high mite levels and subsequent viral load, and had then been robbed by nearby bees. It would make sense that many of these robbers came from the 3 small hive colonies that experienced the dramatic spike in varroa levels as we have seen previously that when a bee is robbing, it provides the perfect stationary target for a mite to climb onboard and be escorted to its new home. Of the 4 colonies of the small hive group that perished over winter, 3 were these colonies that had the high mite level spike. The 4th was a colony whose queen became a drone layer in July 2013 and thus the colony lacked female brood to rear as a new queen, as well as the essential workers of the hive.
Seeley ends this section by saying that he wants to repeat this experiment to verify the results, and intends to space the colonies further apart in order to minimize mite transmission between hives through drifting and robbing behaviour. I hope he does as I believe it will further support his hypothesis that small hives provide great benefits to honey bees.
Living With Smaller vs Larger Comb Cells
For some time, beekeepers have considered whether small cells might help in the battle against varroa mites. The idea is that the reduction in space between the developing bee and the wall of the cell might inhibit the movements of the immature mites, thus disrupting their development and reproductive success. In chapter 5, we saw how the wild colonies living in the Arnot Forest have an average worker cell comb of 5.19 millimeters, which is smaller than the standard beeswax foundation many beekeepers use of 5.38 millimeters. So is it possible that the smaller cell size assists the wild colonies in managing varroa? Sadly, studies have not supported this hypothesis.
Seeley references three studies that looked at worker comb cell size. Researchers compared varroa mite population growth in hive using small cell (4.91mm/0.193in) and standard cell (5.38mm/0.212in), and found no difference. Seeley decided to conduct his own experiment with one of his students, Sean R. Griffin. The pair established 7 pairs of colonies that all had an equal varroa mite level/infestation. Each pair consisted of one hive with small cell and the other with standard. They also removed any drone comb the bees created in order to keep all comb consistently as worker cell only. Despite the clear difference in comb size, no changes in varroa mite infestation levels were detected.
Seeley points out that this isn’t entirely unexpected when you consider the fact that, even in small cell comb, the varroa mite still has amble space to move around on the developing bee. When Seeley and his student (as well as a separate research team in Ireland) examined the ratio of bee thorax width to cell width (known as ‘the fill factor’) for small cell and standard, they found that bees in standard cells had a fill factor of 73% compared to the 79% fill factor of small cell comb. This difference is apparently not enough to inhibit mite movement upon the pupae and thus small cell comb does not appear to function in any mite resistance.
Living With a High vs a Low Nest Entrance
In chapter 5, we learned that wild colonies of honey bees will almost exclusively establish nests high above the ground. This is extremely different to how we maintain our managed hives where the hive (and it’s entrance) are kept low to the ground for our own ease in management. Seeley considers why bees choose such high places to live.
One possibility is the increase in safety when taking cleansing flights in winter. When leaving a hive close to the ground, bees are prone to crashing on the ground where the cold can quickly lead them to be unable to generate the heat needed to engage their flight muscles. It’s also possible that high nest entrances are chosen to avoid the risk of being buried by snow, blocking off air flow and trapping the bees inside. It’s also possible that the high nest cavities receive more sun exposure during the cooler months, helping to maintain the warm microclimate inside that the bees need to survive winter and raise their brood.
Seeley posits that a large advantage to high nest cavities is avoiding predators such as bears. In 2002, Seeley found 8 bee trees in the Arnot Forest, and another 10 in 2011. Since noting their locations, he visited each colony 3 times a year to monitor their survival. These 18 bee trees have been intermittently occupied during the time he has visited them; with new colonies eventually arriving to replace any that died out. Seeley points out that over 16 years of observation, black bears have clearly been present in the forest but only once has he discovered a nest that was attacked by a bear. The tree containing the nest had been blown over durin a storm, which dropped the nest entrance from 10.9 meters/36 feet above the ground to just 1.2 meters/4 feet. It’s unsurprisingly then that an a hungry bear would discover it when it is so conveniently located. What is interesting, however, is that although the tree showed signs of predation by a bear (claw marks around the nest entrance), the colony of honey bees inside survived the onslaught and were in fact still thriving! More importantly for Seeley’s purposes of learning why bees choose such loft homes, however, is the fact that none of the other 17 bee trees were ever detected by bears. In contrast, all of Seeley’s bait hives have been discovered and predated upon at some point, which is why he had to switch to raising them high above the ground. Clearly, nesting high in trees helps protect bees from marauding bears and other terrestrial predators.
Living With vs Without a Propolis Envelope
In chapter 5, we learned that honey bees nesting in the wild will coat the walls, ceiling, and floors with propolis, an antimicrobial plant resin. In managed hives, honey bees do not mirror this behaviour; instead, they tend to use propolis to fill crevices between frames, supers, and the lid. Evidently, honey bees are stimulated to deposit propolis when small cracks and crevices are discovered. In a cavity within a tree, this leads to creating a thick envelope of propolis upon the inner surface of the nest, as well as around the entrance. This makes sense if we consider that cracks and crevices provide a welcome environment to bacteria, which could reach dangerous levels if left untreated. The propolis works to prevent bacteria establishment in any crevices and inhibits its growth.
A number of studies have looked at whether propolis can aid against the growth of you brood diseases found in honey bees: American foulbrood (bacterial) and chalkbrood (fungal). All of these studies found that propolis does work strongly to prohibit these pathogens.
Marla Spivak and her colleagues at the University of Minnesota conducted an experiment that involved comparing the transcription levels of immune-related genes in worker bees living in hives with a propolis coated interior and a hive with an ethanol coating. After just 7 days, those bees living in the propolis coated hives had lower bacterial loads and lower levels of activity of genes involved in insect immune responses compared to those bees living in the ethanol treated hive. This demonstrates that propolis extracts lower the level of bacteria and fungi in the treatment hives compared to the control group.
This same group of researchers also compared colonies with and without a full propolis envelope over a period of 2 years. 12 colonies were stimulated to create a propolis envelope by affixing plastic ‘propolis trap’ material to the inner walls of the hive. The other 12 colonies were left with bare walls and thus their inhabitants did not coat them with propolis. Each year, the researchers measured the health of all 24 colonies.

During the summer and autumn months, it was found that the activity level of several genes involved in insect immunity were consistently lower in the worker bees living in hives with a propolis envelope. This is an important discovery because a highly active immune system is energetically costly for the bee, which can inhibit her activity in other areas such as brood rearing, food collection, comb building, etc. It was also found that those hives with a propolis envelope had greater levels of survival over the 2 year study compared to the hives without. They also consistently had more brood in May, and superior nutritional levels of the young worker bees for both years. Nutritional levels were assessed measuring the level of activity of the gene Vg in both hive groups. Vg is the gene that is activated to produce vitellogenin, which is their primary storage protein. In a healthy nurse bee, 40% of the protein in her body fluids will be vitellogenin because it’s what these young bees use to produce royal jelly to feed to the young larvae. Healthy, well-nourished nurse bees are essential for colony success so this experiment’s results have revealed just how vital the propolis envelope is to a honey bee colony and its overall health.
*
And that's it for this chapter! Next episode, I will be summarizing the final chapter of the book, which is called 'Darwinian Beekeeping'. It goes over all that has been learned throughout the book so far, and then gives recommendations as to how we can use this knowledge to better manage our colonies. I'm excited to get to it!
Hope you are all staying say out there. Thanks so much for reading and listening! And, as always, hug your hens and then wash your hands. Take care and enjoy the Autumn colours!
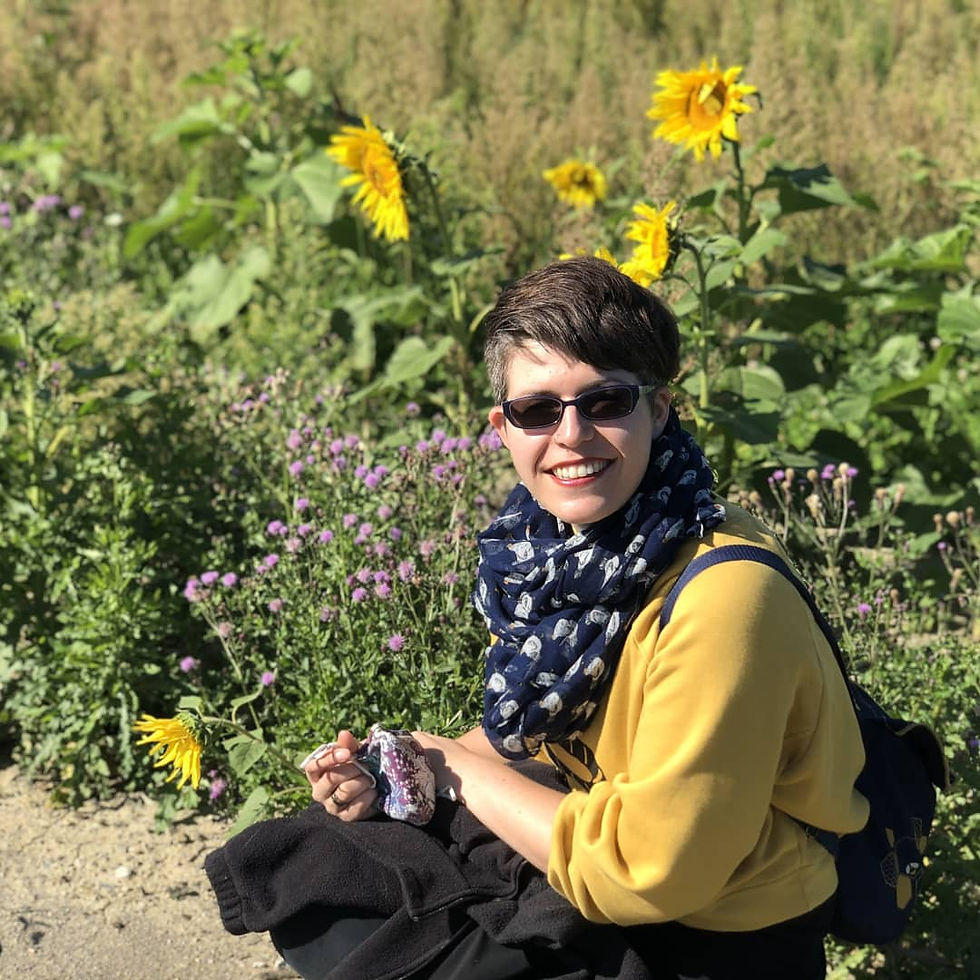
Comentários